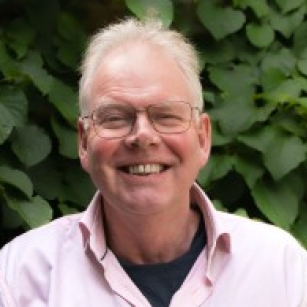
Enzymology and drug side effects
The situation outlined earlier is not isolated. Adverse Drug Reactions, ADRs, are one of the leading causes of hospitalizations and deaths worldwide. It is estimated that after other common causes of mortality such as heart disease, cancer and stroke, ADRs are the fourth most common cause of death. ADRs account for 3-11% of hospitalizations in the United States and Europe, and the health care implications are profound. Not infrequently, patients who suffer serious adverse drug reactions spend several days in an intensive care unit. Although many of these ADRs are preventable and often attributed to human error, genetic causes are suspected in about 50% of ADRs.
Fortunately, genetic variability between humans and the genetic predisposition to side effects of certain drugs is receiving increasing attention and more research is being done, including on drugs used to treat patients with cancer. Enzymology can make an important contribution to preventing these types of side effects. I will explain this in more detail using some examples.
Dihydropyrimidine dehydrogenase
5-fluorouracil (5FU) is a drug that has been used to treat certain tumors since the 1950s. 5FU must first be converted to active metabolites to kill cancer cells, but 80% of administered 5FU is broken down and inactivated by the enzyme dihydropyrimidine dehydrogenase (DPD). However, in patients with a dysfunctional DPD enzyme, more 5FU remains in the body that can be converted to active metabolites and this then also leads to more damage to healthy cells. About 30% of patients receiving 5FU or capecitabine, a related drug, develop serious side effects.
Almost 25 years ago, me and my team showed that DPD deficiency is the main cause of these side effects1. In the years since, we and others have done a lot of research on this, which has resulted in the fact that testing for DPD deficiency before treating patients with these fluoropyrimidines is now recommended by the European Society of Medical Oncology and many regulatory agencies, including the European Medicines Agency.
Pharmacogenetics and the DPD gene
But what is the best way to test whether patients have a DPD deficiency? The simplest and also the best way is to screen for the presence of harmful variants in the DPD gene (DPYD). This genetic testing to see if certain drugs are tolerated or effective is called pharmacogenetics.
Currently, guidelines have been published for four such harmful variants whereby a reduction in the starting dose of 5FU or capecitabine is advised when carrying such a variant in the DPD gene. About eight out of every hundred people in the Dutch population are carriers of one of these four variants and if these people were to receive the full dose of 5FU or capecitabine they run a high risk of becoming seriously ill or even dying from the treatment.
The implementation of this pharmacogenetic test in the Netherlands has therefore been - and still is - enormously important in identifying a large group of patients at increased risk of severe toxicity. These four variants screened for in the Netherlands and in a number of other European countries occur predominantly in individuals with a Northern European background. In individuals with other backgrounds, these four variants sometimes occur very rarely or not at all. When you realize that Amsterdam has about 180 different nationalities, you can imagine that the current method of testing is far from reaching everyone in our society. In addition, many more harmful or potentially harmful variants in the DPD gene are known, but these are often rare and therefore it is impossible to investigate their consequences in a clinical study. Better care is needed here, and in my view, enzymology can play a major role in this.
Better care
In a recent publication, I and my colleagues describe two patients who died as a result of side effects that occurred following the administration of the drug: capecitabine.2
I introduced the first patient at the beginning of my oration. She is a 65-year-old woman with breast cancer and she tested negative for the four variants. Therefore, she received the full dose of capecitabine. After six days, she went to the hospital emergency room with fever and cough, and therapy with capecitabine was discontinued. But by then it was too late: her symptoms quickly worsened, increased in variety, and fourteen days after starting therapy, she died.
The second patient was a 55-year-old man with a malignant tumor in the stomach. This patient was also tested prior to therapy to see if he had any of the four screening variants and this was found to be the case. Therefore, the starting dose was reduced by 50%, in accordance with the advice given in the guidelines. However, after only two days, this patient developed a severe form of diarrhea and therapy with capecitabine was stopped immediately. In the following days, his symptoms worsened, he developed severe inflammation and had almost no white blood cells, which are necessary for your immune system. This patient eventually died a few weeks after starting treatment.
In both patients, DPD activity was only looked at during hospitalization and was found to be completely deficient in both patients. An analysis of a larger portion of the DPD gene showed that the first patient had two other deleterious variants: a known variant, which occurs mainly in the Netherlands, and a second, ultra-rare variant. Both variants lead to a shortened DPD protein, which is therefore inactive. The second patient was found to have a previously undetected, new variant in addition to the screening variant already found. We then made a so-called recombinant DPD enzyme in the laboratory that contained this variant and this mutant DPD enzyme had only 5% residual activity compared to a normal DPD enzyme.
What this study shows is that these two patients died because of rare, harmful variants in the DPD gene, which current, standard genetic testing offered does not detect. And here, enzymology can play an important, sometimes life-saving role. Biochemical phenotyping, such as determining the activity of this DPD enzyme, can help identify patients with DPD deficiency and clarify whether novel structural variants are associated with impaired DPD function. This may prevent the unnecessary death of patients due to DPD deficiency. With enzymology, you know for sure. Enzymology is: knowing for sure!
That this enzymology approach works is shown by the following example. This patient is a 59-year-old woman with colon cancer3. Prior to treatment with capecitabine, among others, she was screened for the four variants and she was found to be a carrier of one of these four variants. This would mean that this patient would have to be treated with 50% of the normal dose. The attending physician at the hospital requested additional analysis of DPD activity just to be sure, and that was just as well, because in our laboratory this patient was found to be completely DPD-deficient. The analysis of the DPD gene then showed a never before reported multiplication of a portion of the DPD gene. This excess DNA prevents a properly functioning DPD enzyme from being formed. This patient was then treated with only 0.8% of the dose normally used for this form of therapy. You can imagine that if this patient had been treated with the planned dose reduction of 50%, she would not have survived.
Customization
Customized care is increasingly common today, but there are still steps we can take here. Standardization and evidence-based medicine have given us many advantages: clinically proven treatments have been standardized and laid down in protocols, so that every patient in the Netherlands has access to them in principle. However, this uniformity also has a downside. Not every patient is the same. For example, a patient's background or the presence of a rare or new variant in their DNA may influence the effect of a drug. With the ever-increasing use of DNA screening strategies that analyze large sections of the genome, the chances of finding new or obscure variants are increasing. The availability of enzymology is crucial here. Enzymology gives direction to genome research: with enzymology, a statement can be made with certainty whether a DNA change leads to impaired enzyme function. By using enzymology within this subfield of pharmacogenetics, we can provide even better care for each individual patient. With enzymology you know for sure!
An important ambition of mine is to develop tools and guidelines on how to deal with new or rare variants in the DPD gene in which enzymology can provide guidance. In the coming years, together with my team and my colleagues from the Department of Medical Oncology and the Pharmacy of the Amsterdam UMC, I will investigate whether a combination of DPD genotyping and measuring DPD enzyme activity before the start of treatment of patients can lead to fewer side effects at a comparable effectiveness.
Enzymology and cancer metabolism
Enzymes not only play an important role in determining the effectiveness of certain drugs in cancer, but they are also essential to the cancer cell itself. Enzymes are protein molecules that act as catalysts in biological reactions, speeding up chemical reactions that would otherwise be too slow to support life processes. In cancer cells, certain enzymes are overactive or mutated, leading to disruptions in normal cell division and growth.
An important aspect of cancer cell metabolism is the Warburg effect, named after Otto Warburg, who discovered that cancer cells exhibit a characteristic change in their metabolism, fermenting glucose into lactate even in the presence of oxygen. Normal cells use oxidative phosphorylation for energy production in oxygen-rich conditions. This aberrant metabolism in cancer cells focuses on producing building blocks for growth, even at the expense of efficient energy production. Enzymes play a crucial role in this reprogramming that allows cancer cells to grow and survive.
Understanding these enzymatic processes provides clues for the development of targeted anti-cancer therapies. By addressing the metabolic dependence of cancer cells, specific and targeted therapies can be developed to treat cancer. However, this requires first knowing which metabolic pathways are essential for a particular type of cancer cell, because one cancer is not the other, and metabolic dependence can differ between different types of cancers and even within a single cancer type.
Neuroblastoma
One particular type of tumor that I work on with my team, along with Professor Tytgat of the Princess Maxima Center, is neuroblastoma. In the Netherlands, nearly 600 children and teenagers up to the age of 18 are diagnosed with cancer every year. Neuroblastoma is a common solid tumor in children and originates from precursor cells of the sympathetic nervous system. In the Netherlands, about 25 children a year are told that they have neuroblastoma. The prognosis for children with neuroblastoma varies widely, ranging from spontaneous remission to a 5-year survival rate of only 50%.
Patients with neuroblastoma are currently treated based on risk groups, which include clinical, pathological and genetic factors. Despite similar treatments, clinical response and survival vary significantly within the same risk group. Therefore, specific and sensitive markers are needed to identify patients at low versus high risk of death and measure response to therapy.
We have shown that a panel of metabolites called catecholamines in urine can confirm whether a patient has neuroblastoma. One catecholamine, namely 3-methoxytyramine, has been shown to be an independent risk factor for poor survival. Elevated 3-methoxytyramine levels in urine indicate an overactive MYCN oncogene in the tumor.4 An oncogene is a gene that, when mutated or overactive, has the potential to transform normal cells into cancer cells. These oncogenes can stimulate cell growth and survival, contributing to cancer development and progression.
The MYC protein plays a key role in the metabolic processes of many cancers, but little is known about the role of this MYC protein and other neuroblastoma-specific genetic traits on metabolism. Several drugs targeting altered metabolic pathways in tumors are in development or already in clinical trials. Together with Professor Tytgat and our teams, I will be identifying important metabolic pathways and enzymes in neuroblastoma patients in the coming years. Understanding their importance can help us unravel the complexity of cancer and this will lead to new therapeutic insights and a tailored treatment strategy for each individual patient. With enzymology, you can be sure. Enzymology is: knowing for sure!
In summary, enzymology contributes to the proper diagnosis and treatment of patients with pharmacogenetic disorders. Applying enzymology can save patients from serious to even deadly side effects of drugs and it enables the development of targeted, new therapies for cancer. So: “Enzymology: be sure!”
References
- Van Kuilenburg et al., Clin Cancer Res (2000) 6, 4705-4712
- Van Kuilenburg et al., JCO Precis Oncol (2024) e2300599
- Henricks et al., Int J Cancer (2018) 142, 424-430
- Verly et al., JCO Precis Oncol (2022) e2000447